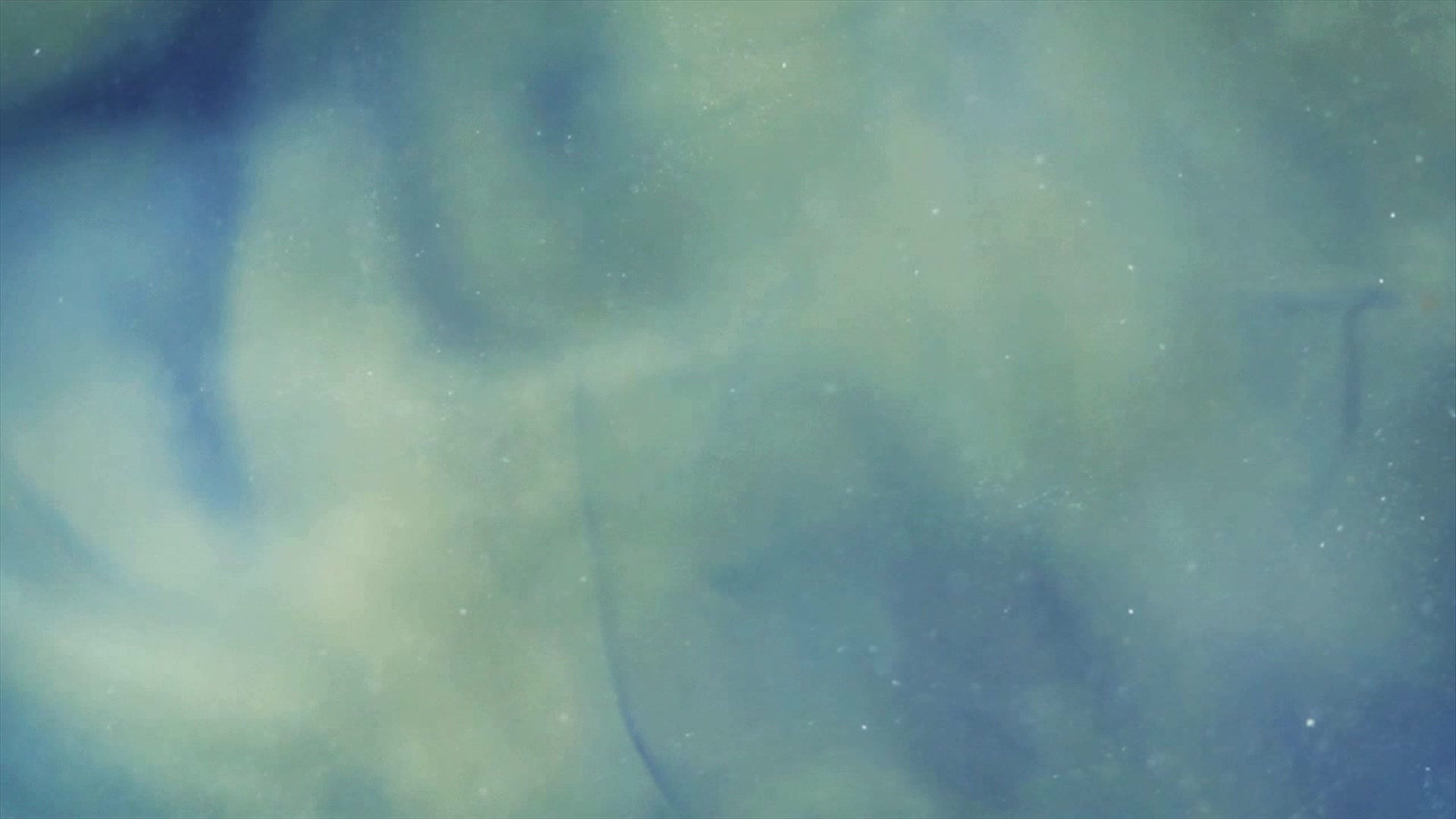
Cosmic Resonator, born from Quantum Physics
Cosmic Resonator is invented based on the knowledge accessible about quantum mechanics. To understand how Cosmic Resonator works, we need to realize that everything in the universe is made out of energy and Cosmic Resonator helps us adjust human's cellular energy levels by utilizing cosmic waves that are very harmonious with our biological wavelength.
Quantum theory is the theoretical basis of modern physics that explains the nature and behavior of matter and energy on the atomic and subatomic level. The nature and behavior of matter and energy at that level is sometimes referred to as quantum physics and quantum mechanics.
Here’s a list of essential elements of quantum physics that everyone ought to know, at least in broad outlines:
1) Particles are waves, and vice versa. Quantum physics tells us that every object in the universe has both particle-like and wave-like properties. It’s not that everything is really waves, and just sometimes looks like particles, or that everything is made of particles that sometimes fool us into thinking they’re waves. Every object in the universe is a new kind of object– call it a “quantum particle” that has some characteristics of both particles and waves, but isn’t really either. Quantum particles behave like particles, in that they are discrete and (in principle) countable. Matter and energy come in discrete chunks, and whether you’re trying to locate an atom or detect a photon of light, you will find it in one place, and one place only. Quantum particles also behave like waves, in that they show effects like diffraction and interference. If you send a beam of electrons or a beam of photons through a narrow slit, they will spread out on the far side. If you send the beam at two closely spaced slits, they will produce a pattern of alternating bright and dark spots on the far side of the slits, as if they were water waves passing through both slits at once and interfering on the other side. This is true even though each individual particle is detected at a single location, as a particle.
2) Quantum states are discrete. The “quantum” in quantum physics refers to the fact that everything in quantum physics comes in discrete amounts. A beam of light can only contain integer numbers of photons– 1, 2, 3, 137, but never 1.5 or 22.7. An electron in an atom can only have certain discrete energy values– -13.6 electron volts, or -3.4 electron volts in hydrogen, but never -7.5 electron volts. No matter what you do, you will only ever detect a quantum system in one of these special allowed states.
3) Probability is all we ever know. When physicists use quantum mechanics to predict the results of an experiment, the only thing they can predict is the probability of detecting each of the possible outcomes. Given an experiment in which an electron will end up in one of two places, we can say that there is a 17% probability of finding it at point A and an 83% probability of finding it at point B, but we can never say for sure that a single given electron will definitely end up at A or definitely end up at B. No matter how careful we are to prepare each electron in exactly the same way, we can never say for definitively what the outcome of the experiment will be. Each new electron is a completely new experiment, and the final outcome is random.
4) Measurement determines reality. Until the moment that the exact state of a quantum particle is measured, that state is indeterminate, and in fact can be thought of as spread out over all the possible outcomes. After a measurement is made, the state of the particle is absolutely determined, and all subsequent measurements on that particle will return produce exactly the same outcome. This seems impossible to believe– it’s the problem that inspired Erwin Schrodinger's (in)famous thought experiment regarding a cat that is both alive and dead– but it is worth reiterating that this is absolutely confirmed by experiment. The double-slit experiment mentioned above can be thought of as confirmation of this indeterminacy– until it is finally measured at a single position on the far side of the slits, an electron exists in a superposition of both possible paths. The interference pattern observed when many electrons are recorded one after another is a direct consequence of the superposition of multiple states. The Quantum Zeno Effect is another example of the effects of quantum measurement: making repeated measurements of a quantum system can prevent it from changing its state. Between measurements, the system exists in a superposition of two possible states, with the probability of one increasing and the other decreasing. Each measurements puts the system back into a single definite state, and the evolution has to start over. The effects of measurement can be interpreted in a number of different ways– as the physical “collapse” of a wave function, as the splitting of the universe into many parallel worlds, etc.– but the end result is the same in all of them. A quantum particle can and will occupy multiple states right up until the instant that it is measured; after the measurement it is in one and only one state.
5) Quantum correlations are non-local. One of the strangest and most important consequences of quantum mechanics is the idea of “entanglement.” When two quantum particles interact in the right way, their states will depend on one another, no matter how far apart they are. You can hold one particle in Princeton and send the other to Paris, and measure them simultaneously, and the outcome of the measurement in Princeton will absolutely and unequivocally determine the outcome of the measurement in Paris, and vice versa. The correlation between these states cannot possibly be described by any local theory, in which the particles have definite states. These states are indeterminate until the instant that one is measured, at which time the states of both are absolutely determined, no matter how far apart they are. This has been experimentally confirmed dozens of times over the last thirty years or so, with light and even atoms, and every new experiment has absolutely agreed with the quantum prediction. It must be noted that this does not provide a means of sending signals faster than light– a measurement in Paris will determine the state of a particle in Princeton, but the outcome of each measurement is completely random. There is no way to manipulate the Parisian particle to produce a specific result in Princeton. The correlation between measurements will only be apparent after the fact, when the two sets of results are compared, and that process has to take place at speeds slower than that of light.
6) Everything not forbidden is mandatory. A quantum particle moving from point A to point B will take absolutely every possible path from A to B, at the same time. This includes paths that involve highly improbable events like electron-positron pairs appearing out of nowhere, and disappearing again. The full theory of quantum electro-dynamics (QED) involves contributions from every possible process, even the ridiculously unlikely ones. It’s worth emphasizing that this is not some speculative mumbo-jumbo with no real applicability. A QED prediction of the interaction between an electron and a magnetic field correctly describes the interaction to 14 decimal places. As weird as the idea seems, it is one of the best-tested theories in the history of science.
7) Quantum physics is not magic. Yeah, this was on the other list as well, but it’s so important that it needs repeating. As strange as quantum physics is– and don’t get me wrong, it’s plenty weird– it does not suspend all the rules of common sense. The bedrock principles of physics are still intact: energy is still conserved, entropy still increases, nothing can move faster than the speed of light. You cannot exploit quantum effects to build a perpetual motion machine, or to create telepathy or clairvoyance.
SOURCE: http://chadorzel.com/principles/2010/01/20/seven-essential-elements-of-qu/